Welcome to Unit 4: Chemical Bonding! Use the gallery below to read about important topics like the ionic bonding, polyatomic ions, covalent bonding, Lewis Structures, octet rule exceptions, the VSEPR Theory, polar molecules, and metallic bonding. Each image contains a description of the concept along with a link to a video about the topic.
Prefer to jump straight into the videos? Click here for a video gallery about these topics.
If you love the images below and would like to customize them for your classroom, they are available for purchase as an editable PowerPoint. You can find them at my site’s shop or my TeachersPayTeachers page. Prices are the same in both places so that you can shop wherever you feel most comfortable.

Ionic Bonding

Properties of Ionic Bonds

Naming Compounds Flow Chart

Naming Ionic Compounds
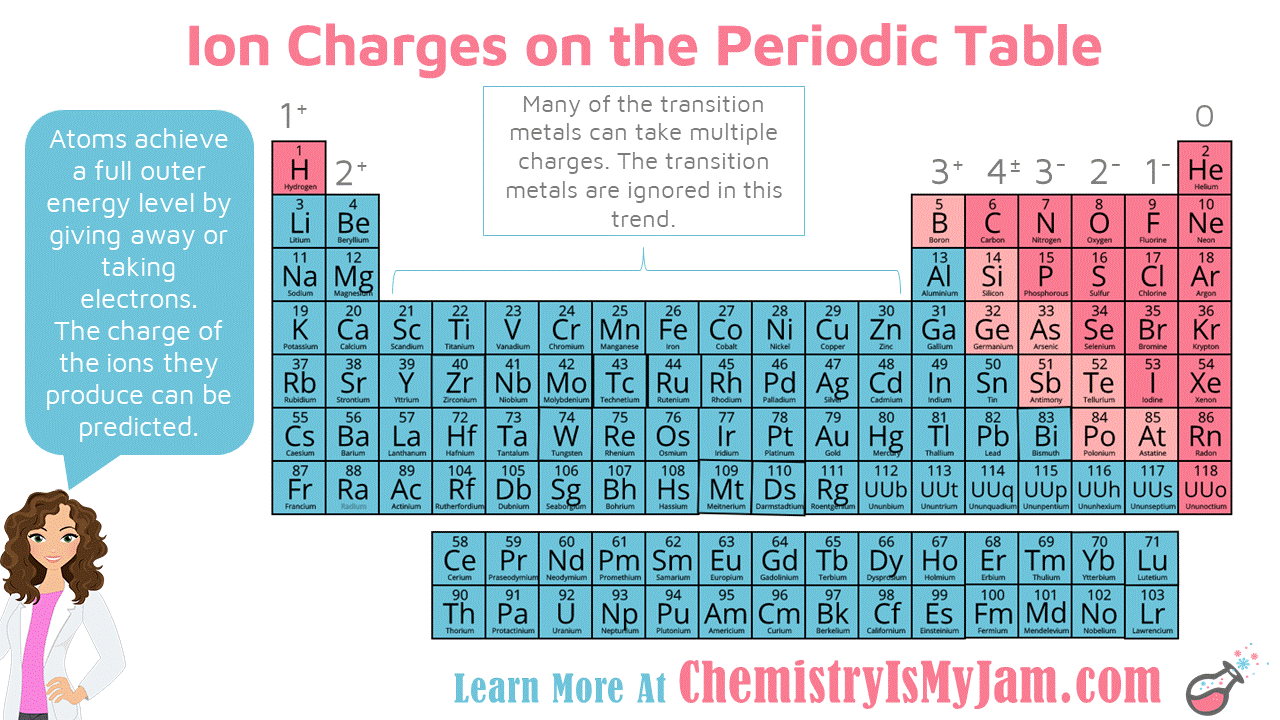
Ion Charges on the Periodic Table

Writing Formulas for Ionic Compounds

Reduce the Formulas for Ionic Compounds

Naming Ionic Compounds With Transition Metals

Writing Formulas for Ionic Compounds With Transition Metals

Polyatomic Ions

Common Polyatomic Ions - The "ates"

The Trend in Polyatomic Ion Names

Covalent Bonding

Covalent Bonding

Naming Molecular Compounds

Writing Formulas for Molecular Compounds

Ionic Versus Molecular Compounds
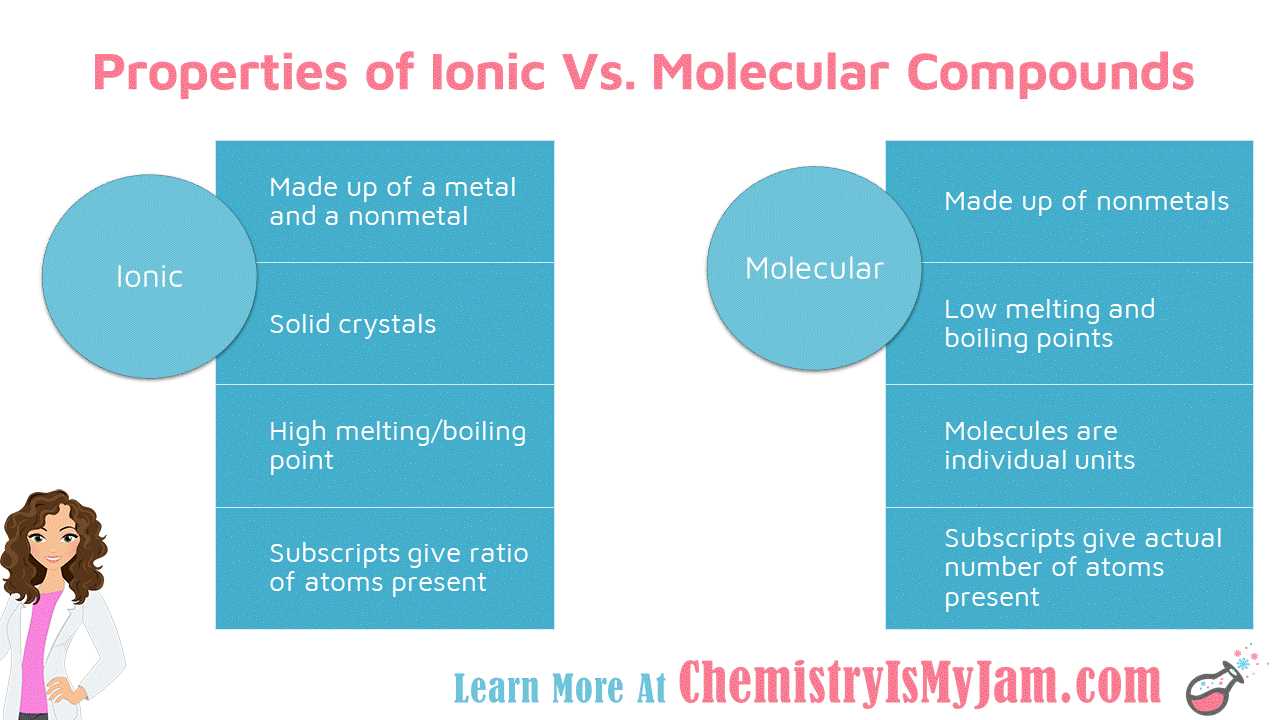
Properties of Ionic and Molecular Compounds
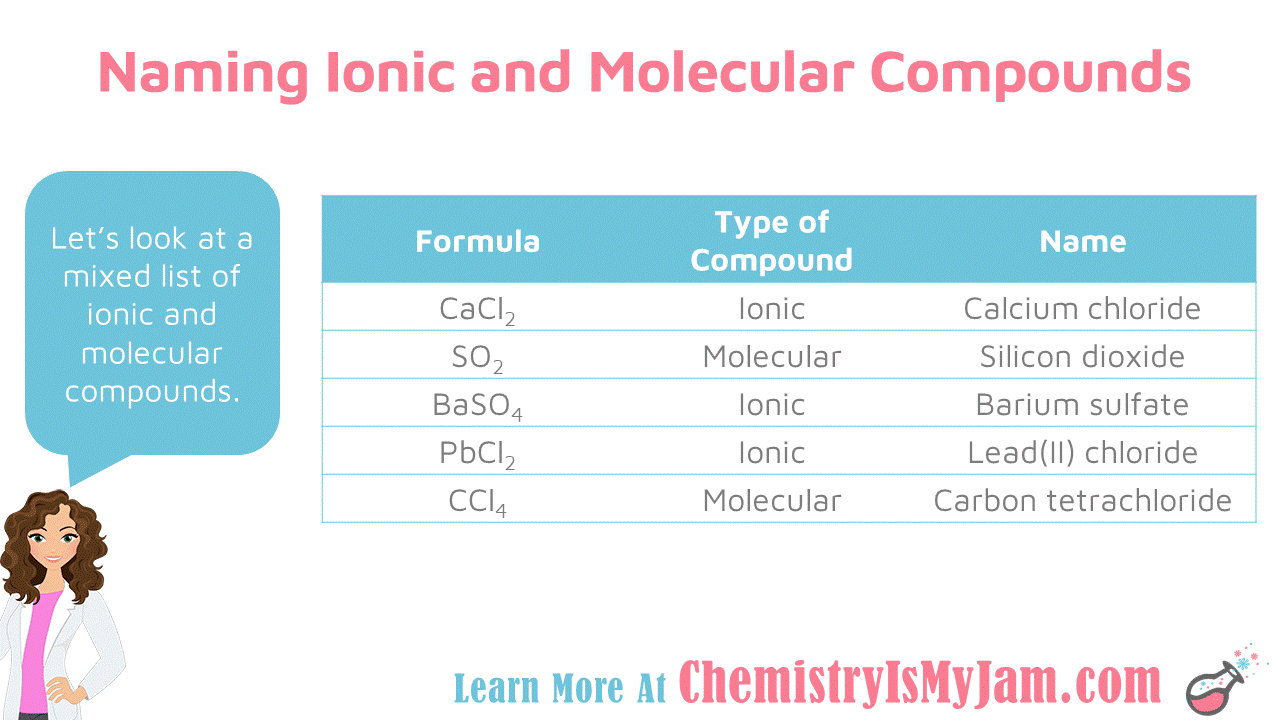
Naming Ionic and Molecular Compounds

Lewis Structure Rules

Lewis Structure for Water

Lewis Structure for Carbon Dioxide

Lewis Structure for the Carbonate Ion

Comparing Covalent Bonds

Octet Rule Exceptions

Lewis Structure for boron trifluoride

Lewis Structure for sulfur hexafluoride

Lewis Structure for sulfur tetrafluoride

VSEPR Chart - 2 and 3 Domains

VSEPR Chart - 4 Domains

Hybridization

Hybridization

Polar Covalent Bonds

Polar Molecules

Metallic Compounds

Substitutional Versus Interstitial Alloys
-
1
Ionic Bonding
Atoms bond in order to achieve full outer energy levels. In the case of ionic bonding, electrons will be transferred. Consider sodium and chlorine. Sodium has 1 electron in it’s outermost energy level, while chlorine has 7. Sodium can give chlorine it’s 1 valence electron. This causes the original outer energy level of sodium to be empty, leaving the next energy level down to be the now full outer energy level. This also leaves sodium with a +1 charge. When chlorine receives the electron, it then has a full outer energy level and a charge of -1. An ionic bond will form between the sodium and chloride ions because they are oppositely charged. Ionic bonds are forces of attraction between oppositely charged particles. -
0
Properties of Ionic Bonds
The key to forming an ionic bond is that an electron is transferred. Ionic bonds are usually made up of elements from opposite sides of the periodic table. They will contain a metal and a nonmetal. After the electron has been transferred, the metal will have a positive charge and the nonmetal will have a negative charge. These oppositely charged particles will form an ionic bond. Compounds formed from ionic bonds are usually solid crystals at room temperature, they have high melting temperature, and they can conduct electric current when dissolved in water. The ions making up an ionic bond will generally form a lattice where oppositely charged particles are held close together. The lattice pictured could be for a compound like sodium chloride (NaCl) where there is a 1:1 ratio between the positive and negative ions. -
2
Naming Compounds Flow Chart
When naming compounds, there are a series of questions you should ask yourself. Begin by asking, “Is there a metal?” If there is a metal, the compound is likely ionic. Then ask yourself, “Does the compound contain a transition metal, Sn, or Pb?” If it does, list the name of the cation, followed by the charge of the cation in roman numerals in parenthesis, followed by the name of the anion ending in “ide.” If there is not a transition metal, Sn, or, Pb, simply list the name of the cation, followed by the name of the anion ending in “ide.” If there was not a metal present, the compound is molecular. Prefixes would be used to indicate the number of each atom present. -
1
Naming Ionic Compounds
Binary ionic compounds are only made up of two elements, a metal and a nonmetal. When naming binary ionic compounds, list the name of the cation, followed by the name of the anion ending in “ide.” For example, NaCl is sodium chloride, Li2O is lithium oxide, and KI is potassium iodide. -
4
Ion Charges on the Periodic Table
Atoms achieve a full outer energy level by giving away or taking electrons. The charge of the ions they produce can be predicted. Take nitrogen for example. Nitrogen has 5 valence electrons. The easiest way for nitrogen to achieve a full outer energy level is to take 3 electrons from another atom, causing nitrogen to have a -3 charge. In the case of magnesium, which has 2 valence electrons, it is easier to give away the 2 electrons than to take 6 from another atom. This causes magnesium to have a +2 charge when it becomes an ion. As you go from left to right across the periodic table, the chares are +1, +2, +3, +/-4, -3, -2, -1, 0. This trend excludes the transition metals because these atoms can take multiple charges and do not follow the normal trend. -
2
Writing Formulas for Ionic Compounds
The charges in an ionic compound should balance out to zero. This explains why the subscripts are necessary in a chemical formula. In the example of lithium oxide, lithium will have a charge of +1 when it becomes an ion. Oxygen will have a charge of -2 when it becomes an ion. Two lithiums are necessary to balance out 1 oxygen. This gives the overall compound a charge of 0. A trick to figuring out the subscripts is to write the charges above the elements and then cross them down. Only cross down the number, not the + or -. The 1+ charge of lithium caused there to be 1 oxygen in the compounds. The 2- charge of oxygen caused there to be 2 lithiums in the compound. The charges written above the compound are not part of the final formula. The final formula is Li2O. -
2
Reduce the Formulas for Ionic Compounds
When writing formulas for ionic compounds, the number should be reduced as much as possible. When you cross down the charges of magnesium and oxygen, it may be tempting to write Mg2O2, however these numbers should be reduced to MgO. The charges for aluminum and phosphorus are 3+ and 3-, however the formula gets reduced to AlP. The charge for calcium is 2+ while the charge for phosphorus is 2-. Crossing down the charges would give a formula of Ca2(SO4)2, but this should be reduced to CaSO4. -
1
Naming Ionic Compounds With Transition Metals
Since transition metals can take multiple charges, chemists needed a way of knowing what charge it was taking in each compound. They used Roman numerals to indicate the charge of the metal. It is often necessary to use the charge of the anion to figure out the charge of the transition metal. As a reminder the roman numerals for numbers 1-6 are: I, II, III, IV, V, and VI. Iron is a transition metal, meaning that it’s charge should be indicated in roman numerals when writing the name of the compound. The charge of oxygen is 2- and there are 3 oxygens. This means that there is a total negative charge of 6- (2- times 3). The compound must have a total positive charge of 6+ to balance out the negative charge. This positive charge is divided by the 2 iron ions that are present, meaning each iron ion has a charge of 3+. The name of the compound would be iron(III) oxide. -
0
Writing Formulas for Ionic Compounds With Transition Metals
When transition metals, Sn, and Pb are present, the charge of the cation should be indicated by roman numerals. In the case of iron(II) oxide, iron has a charge of +2 (from the roman numeral) and oxygen is -2 (it’s normal charge). Cross these down and reduce to get FeO. Chromium(III) nitrate has a chromium with a charge of +3 (from the roman numeral) and nitrate with it’s normal -1 charge. Cross these down to get Cr(NO3)3. Lead(II) sulfide has lead with a charge of +2 and sulfur with a charge of -2. Cross the charges down and reduce to get PbS. A few exceptions: Silver will always be Ag1+, zinc will always be Zn2+, and cadmium will always be Cd2+. It is not necessary to use roman numerals for these. -
0
Polyatomic Ions
Polyatomic ions are groups of atoms that stay together and have a charge. Break down the word into it’s parts. “Poly” means many. “Ion” means atom with a charge. Polyatomic ions are many atoms that have a charge. Whenever an ionic compound has more than 2 elements, a polyatomic ion is present. Nitrate is an example of a common polyatomic ion. Nitrate will always consist of 1 nitrogen atom and 3 oxygen atoms bonded together. All of the atoms combined have a charge of 1-. The nitrate ion will always have a charge of 1-. An interesting thing to note about polyatomic ions: the bonds between the nitrogen and oxygen atoms are covalent bonds. The group of atoms together will then form ionic bonds with other ions. So there are covalent bonds within the polyatomic ion but then it ionically bonds to other ions. -
1
Common Polyatomic Ions - The "ates"
It is often helpful to memorize the polyatomic ions. One method is to memorize the “ate” version of the ions. If you know the “ate” version, you can easily convert this to the other versions of the ion by changing the number of oxygens (shown next). The most common “ate” polyatomic ions are listed here. They are arranged in order of decreasing charge. Bromate is BrO3-, chlorate is ClO3-, nitrate is NO3-, sulfate is SO42-, carbonate is CO32-, and phosphate is PO43-. -
1
The Trend in Polyatomic Ion Names
It is often helpful to memorize the polyatomic ions. One method is to memorize the “ate” version of the ions. If you know the “ate” version, you can easily convert this to the other versions of the ion by changing the number of oxygens. Adding 1 oxygen to the “ate” version of the ion turns it into a “per____ate” ion. Subtracting one oxygen from the “ate” version of the ion turns it into the “ite” ion. Subtracting 2 oxygens from the “ate” version of the ion turns it into the “hypo____ite” version of the ion. An example of this is the chlorate ion. The “ate” version of the ion is ClO3-. Adding an oxygen gives ClO4- which is the perchlorate ion. Subtracting an oxygen from chlorite gives ClO2-, which is the chlorite ion. Subtracting 2 oxygens from chlorate gives ClO-, which is the hypochlorite ion. -
0
Covalent Bonding
Atoms bond in order to achieve full outer energy levels. In the case of covalent bonding, electrons are shared. Consider the fluorine atom. Fluorine has 7 valence electrons. Fluorine would have a full outer energy level with 8 valence electrons. Two fluorine atoms can share two electrons (one electron from each atom). By sharing 2 electrons, each fluorine has a full outer energy level. This is an example of a covalent bond. -
0
Covalent Bonding
The key to covalent bonding is that electrons are shared. Covalent bonds are formed between two nonmetals atoms. Covalent compounds have low melting and boiling points. They have poor electrical conductivities. Covalent bonding forms molecules, or molecular compounds. These are individual units, unlike ionic compounds which form a crystal structure. -
0
Naming Molecular Compounds
When naming molecular compounds, prefixes are added at the beginning of each element to tell the number of each element present in the molecule. The name of the molecule will still end in “ide.” “Mono” can be omitted if there is only one of the first element, but it cannot be omitted from the second element. Charges are not necessary for writing the formulas of molecular compounds. The formula for molecular compounds represents the actual number of atoms present and should not be reduced to the lowest possible form. It is necessary to memorize the prefixes in order to name molecular compounds. The prefix for 1 is mono, 2 is di, 3 is tri, 4 is tetra, 5 is penta, 6 is hexa, 7 is hepta, 8 is octa, 9 is nona, and 10 is deca. -
0
Writing Formulas for Molecular Compounds
When writing formulas for molecular compounds, the prefixes before each element tell the number of atoms of that element present. The prefix for 1 is mono, 2 is di, 3 is tri, 4 is tetra, 5 is penta, 6 is hexa, 7 is hepta, 8 is octa, 9 is nona, and 10 is deca. Dinitrogen pentoxide is N2O5, carbon dioxide is CO2, and carbon monoxide is CO. Note that “mono” can be omitted from the first element in a compound, but not from the second. -
0
Ionic Versus Molecular Compounds
Ionic compounds form crystal structures of repeating ions. The formula for an ionic compound will indicate the ratio of each atom, meaning the formula has been reduced to it’s lowest possible form. NaCl for example means that the sodium and chloride ions are in a 1:1 ration – not that there is only one of each present. Molecular compounds are individual units. The formula gives the actual number of atoms present in the molecule. Water for example (H2O) is always made up of 2 hydrogen atoms and 1 oxygen atom. The formulas for molecular compounds are not reduced to their lowest possible form. -
0
Properties of Ionic and Molecular Compounds
Ionic compounds are made up of a metal and a nonmetal. They are generally solid crystals at room temperature. They have high melting and boiling points. When writing the formulas for ionic compounds, it is necessary to cross down the charges of the ions to create a neutral compound. The subscripts give a ratio of atoms present. Molecular compounds are made up of nonmetals. They have low melting and boiling points. Molecules are individual units. The subscripts indicate the actual number of atoms present. It is not necessary to cross down charges when writing formulas. -
0
Naming Ionic and Molecular Compounds
Let’s examine a mixed list of ionic and molecular compounds. We will determine if they are ionic or molecular and then name them. CaCl2 contains a metal, making it an ionic compound. To name a simple ionic compound, list the name of the cation, followed by the name of the anion ending in “ide.” This is calcium chloride. SO2 does not contain a metal, making it a molecular compound. Prefixes should be used to indicate the number of each atom present. “Mono” can be left off of the first element, but not the second element. This is silicon dioxide. BaSO4 is an ionic compound because it contains a metal, and because it has a polyatomic ion. When a compound ends in a polyatomic ion, the compound will not end in “ide” but instead will end with the name of the polyatomic ion. This is barium sulfate. PbCl2 is ionic because it contains a metal. Lead is one of the elements that can take multiple charges, meaning it’s charge needs to be listed in Roman numerals. Use the charge of chlorine (1-) to determine the charge of lead (2+). This is lead(II) chloride. CCl4 is molecular because it does not contain a metal. This is carbon tetrachloride. -
1
Lewis Structure Rules
Lewis Structures are diagrams that show the bonding between the atoms in a molecule, as well as the valence electrons. There steps for drawing Lewis Structures are: Total the number of valence electrons. Positive charges should be subtracted from the total (because they indicate that electrons have been lost) and negative charges should be added to the total (because they indicate that electrons have been gained). Arrange the atoms with carbon or the least prevalent atom in the center. Place bonds connecting the atoms. Complete the octets by adding electrons. Remember that bonds count as two electrons. There are a couple of exceptions to the octet rule. Hydrogen can only have 2 electrons. This means hydrogen can handle having one bond, but that is it. No other electrons should be added to hydrogen. Boron is often stable with 6 electrons rather than 8. Compare what you have drawn to the number of valence electrons from step 1. If you have drawn too many electrons, make double or triple bonds. Each time you make a double or triple bond, a pair of electrons is remove from both atoms that the bond touches. If you have not drawn enough electrons, extra electrons go onto the central atom. -
0
Lewis Structure for Water
To determine the Lewis Structure of water, first total the number of valence electrons. There are 2 hydrogens, each with 1 valence electron. There is 1 oxygen with 6 valence electrons, giving a total of 8 valence electrons. Arrange the atoms with the least prevalent atom in the center. This puts oxygen in the center with a hydrogen on each side. Complete the octets for each atom. A bond counts as 2 electrons for each atom. The two bonds on oxygen give it 4 electrons. Four more electrons needed to be added to oxygen to complete the octet. Hydrogen can only handle two electrons, meaning no additional electrons should be added to the hydrogens. Compare the number of electrons you have drawn to the number of valence electrons you initially calculated. Recall that bonds count as two electrons each. This Lewis Structure for water has 8 electrons, which matches the number of valence electrons. As long as all atoms have the correct number of electrons and the total electrons match the valence electrons, you have a good Lewis Structure. -
0
Lewis Structure for Carbon Dioxide
To determine the Lewis Structure of carbon dioxide, first total the number of valence electrons. There are 2 oxygens, each with 6 valence electrons. There is 1 carbon with 4 valence electrons, giving a total of 16 valence electrons. Arrange the atoms with the least prevalent atom in the center. This puts carbon in the center with an oxygen on each side. Complete the octets for each atom. A bond counts as 2 electrons for each atom. The two bonds on carbon give it 4 electrons. Four more electrons needed to be added to oxygen to complete the octet. The bond for oxygen gives it 2 electrons, meaning that each oxygen needs an additional 6 electrons. Compare the number of electrons you have drawn to the number of valence electrons you initially calculated. Recall that bonds count as two electrons each. This Lewis Structure for carbon dioxide has 20 electrons, which is higher than the number of valence electrons. This means that double bonds need to be created. Any time you make a double bond, erase two electrons from each atom that participates in the bond. Each time you create a double bond, the total number of electrons should drop by 2. By creating 2 double bonds, the total number of electrons dropped from 20 to 16, which matches the number of valence electrons. Carbon dioxide should have two double bonds. As long as all atoms have the correct number of electrons and the total electrons match the valence electrons, you have a good Lewis Structure. -
0
Lewis Structure for the Carbonate Ion
To determine the Lewis Structure of the carbonate ion (CO32-), first total the number of valence electrons. There are 3 oxygens, each with 6 valence electrons. There is 1 carbon with 4 valence electrons, giving a total of 22 valence electrons. The ion has a charge of -2, meaning that 2 more electrons should be added to the total. This ion has 24 valence electrons. Arrange the atoms with the least prevalent atom in the center. This puts carbon in the center with an oxygen on each side. Complete the octets for each atom. A bond counts as 2 electrons for each atom. The three bonds on carbon give it 6 electrons. Two more electrons needed to be added to carbon to complete the octet. The bond for oxygen gives it 2 electrons, meaning that each oxygen needs an additional 6 electrons. Compare the number of electrons you have drawn to the number of valence electrons you initially calculated. Recall that bonds count as two electrons each. This Lewis Structure for the carbonate ion has 26 electrons, which is higher than the number of valence electrons. This means that double bonds need to be created. Any time you make a double bond, erase two electrons from each atom that participates in the bond. Each time you create a double bond, the total number of electrons should drop by 2. By creating 1 double bond, the total number of electrons dropped from 26 to 24, which matches the number of valence electrons. The carbonate ion should have one double bond. As long as all atoms have the correct number of electrons and the total electrons match the valence electrons, you have a good Lewis Structure. Lewis structures for ions are often put in brackets with the charge on the outside of the brackets. -
0
Comparing Covalent Bonds
Bond strength is a measure of the energy required to break a bond. Covalent bonds can be single (meaning 2 electrons are shared), double (meaning 4 electrons are shared), or triple (meaning 6 electrons are shared). Single bonds are the longest and the weakest. Triple bonds are the shortest and the strongest. -
1
Octet Rule Exceptions
Typically atoms have 8 valence electrons around them when they are bonded together, and this is called the octet rule. There are two types of octet rule exceptions. The first type is when atoms have less than 8 valence electrons. The two most common examples of this are hydrogen and boron. Hydrogen can only have 2 valence electrons and boron is often satisfied with 6. The second type of octet rule exception is when atoms have more than 8 electrons. The elements in period 3 and below have an empty d shell that can be used to house extra electrons. This is often referred to as an “expanded octet.” -
0
Lewis Structure for boron trifluoride
To determine the Lewis Structure of the boron trifluoride (BF3), first total the number of valence electrons. There are 3 fluorines, each with 7 valence electrons. There is 1 boron with 3 valence electrons, giving a total of 24 valence electrons. Arrange the atoms with the least prevalent atom in the center. This puts boron in the center with 3 fluorines around it. Complete the octets for each atom. A bond counts as 2 electrons for each atom. The four bonds on boron give it 6 electrons. Recall that boron is often satisfied with only 6 electrons. No additional pairs of electrons are needed. The bond for each fluorine gives it 2 electrons, meaning that each fluorine needs an additional 6 electrons. Compare the number of electrons you have drawn to the number of valence electrons you initially calculated. Recall that bonds count as two electrons each. This Lewis Structure for the boron trifluoride molecule has 24 electrons, which matches the number of valence electrons. This is an octet rule exception because the boron has less than 8 electrons. This is common for boron and is a correct Lewis Structure. -
0
Lewis Structure for sulfur hexafluoride
To determine the Lewis Structure of the sulfur hexafluoride (SF6), first total the number of valence electrons. There are 6 fluorines, each with 7 valence electrons. There is 1 sulfur with 6 valence electrons, giving a total of 48 valence electrons. Arrange the atoms with the least prevalent atom in the center. This puts sulfur in the center with 6 fluorines around t. Complete the octets for each atom. A bond counts as 2 electrons for each atom. The four bonds on sulfur give it 8 electrons. The bond for each fluorine gives it 2 electrons, meaning that each fluorine needs an additional 6 electrons. Compare the number of electrons you have drawn to the number of valence electrons you initially calculated. Recall that bonds count as two electrons each. This Lewis Structure for the sulfur hexafluoride molecule has 48 electrons, which matches the valence electrons. By adding the extra bonds to sulfur, sulfur has exceeded the octet rule. This is allowed as long as sulfur is in period 3 or below on the periodic table. This is called an expanded octet. Sulfur has used it’s empty d sublevel to house the extra electrons. -
0
Lewis Structure for sulfur tetrafluoride
To determine the Lewis Structure of the sulfur tetrafluoride (SF4), first total the number of valence electrons. There are 4 fluorines, each with 7 valence electrons. There is 1 sulfur with 6 valence electrons, giving a total of 34 valence electrons. Arrange the atoms with the least prevalent atom in the center. This puts sulfur in the center with a fluorine on each side. Complete the octets for each atom. A bond counts as 2 electrons for each atom. The four bonds on sulfur give it 8 electrons. The bond for each fluorine gives it 2 electrons, meaning that each fluorine needs an additional 6 electrons. Compare the number of electrons you have drawn to the number of valence electrons you initially calculated. Recall that bonds count as two electrons each. This Lewis Structure for the sulfur tetrafluoride molecule has 32 electrons, which is lower than the number of valence electrons. When extra electrons are needed, they should go on the central atom. By adding the extra electrons to sulfur, sulfur has exceeded the octet rule. This is allowed as long as sulfur is in period 3 or below on the periodic table. This is called an expanded octet. Sulfur has used it’s empty d sublevel to house the extra electrons. -
0
VSEPR Chart - 2 and 3 Domains
A VSEPR Chart helps you relate the number of domains (regions of space where pairs of electrons are located) to the shape of molecules. It is helpful to have a Lewis Structure drawn when consulting a VSEPR chart. Focus on the central atom in the Lewis Structure. CO2 has two double bonds coming off of the central atom. Each double bond is one domain. CO2 has a total of 2 domains with 0 lone pairs. This is a linear molecule. BH3 has three single bonds coming off of the central atom, each counting as one domain. BH3 has a total of 3 domains with 0 lone pairs. The shape is trigonal planar. SO2 has a single bond, a double bond, and a lone pair on the central atom, each counting as one domain. SO2 has 3 domains with 1 lone pair giving it a bent shape. Notice that the pair of electrons on the sulfur do not show up in the picture of the molecule, however the do take up space and affect the shape of the molecule. -
1
VSEPR Chart - 4 Domains
A VSEPR Chart helps you relate the number of domains (regions of space where pairs of electrons are located) to the shape of molecules. It is helpful to have a Lewis Structure drawn when consulting a VSEPR chart. Focus on the central atom in the Lewis Structure. CH4 has four double bonds coming off of the central atom, each counting as one domain. CH4 has a total of 4 domains with 0 lone pairs. This molecule has a tetrahedral shape. NH3 has three single bonds and a pair of electrons coming off of the central atom, each counting as one domain. NH3 has a total of 4 domains with 1 lone pair. The shape is trigonal pyramidal. Notice that the pair of electrons on the nitrogen do not show up in the picture of the molecule, however the do take up space and affect the shape of the molecule. H2O has 2 single bonds and 2 lone pairson the central atom, each counting as one domain. H2O has 4 domains with 2 lone pairs giving it a bent shape. -
0
Hybridization
Orbitals within an atom mix, or hybridize, to form hybrid orbitals that better allow for bonding to take place. The picture shows regular atomic orbitals on the left and hybridized orbitals on the right. The regular atomic orbitals are the 2s and three 2p orbitals. The s orbital and one of the p orbtials have hybridized to form a 2sp orbital. There are also 2 unhybridized p orbitals remaining on the right hand side. Notice that the number of orbtials you start with matches the number or orbitals you end up with. Also notice that the energy of the hybridized 2sp orbital is lower than the original energy of the p orbitals, making this a favorable situation. -
0
Hybridization
The type of hybridization taking place can be determined by counting the total number of domains. Domains are regions of space around the central atom where pairs of electrons are located. A molecule with 2 domains will have an sp hybridized orbital and a bond angle of 180 degrees. CO2 is an example of an sp hybridized carbon. A molecule with 3 domains will have sp2 hybridized orbitals and a bond angle of 120 degrees. BH3 is an example of an sp hybridized carbon. A molecule with 4 domains will have sp3 hybridized orbitals and a bond angle of 109.5 degrees. NH3 is an example of an sp hybridized carbon. Note that these bond angles are estimates. Non-bonded pairs of electrons take up slightly more space than bonded electrons causing there to be slight variations in these numbers. -
0
Polar Covalent Bonds
Polar bonds have a partially positive end and a partially negative end. This is caused by differences in electronegativity. The element with the higher electronegativity pulls the electrons closer to itself. The bond between hydrogen and chlorine in HCl is a polar bond. There is a large electronegativity difference between hydrogen and chlorine. The bond between the two chlorines in Cl2 is a nonpolar bond. There is no electronegativity difference between chlorine and chlorine. Indicate polar bonds with an arrow with a line through the end. The arrow points at the more electronegative element. It is also common practice to write δ+ above the less electronegative atom and δ- above the more electronegative atom. The δ symbol “delta” means partially charged. -
0
Polar Molecules
Not all molecules with polar bonds are polar molecules. The polarity of bonds can be cancelled out by symmetry resulting in a nonpolar molecule. This is common in linear, planar, and tetrahedral molecules. Carbon dioxide is a linear molecule. The two oxygens on the outside have higher electronegativity values than the carbon in the middle. This causes there to be partially negative regions of space on the oxygens and a partially positive region in the center. Because this molecule is so symmetric, the polarity of the molecule gets cancelled out. Even though there are polar bonds present, the molecule itself is nonpolar. When evaluating polar molecules, be on the lookout for symmetry, which can cancel out the polarity of the bonds. -
0
Metallic Compounds
In metallic compounds, valence electrons are delocalized. This means that the valence electrons are not bound to one atom. They move freely between all of the atoms present. This is often called a sea of electrons. Many metallic properties can be explained by delocalized electrons. Metals are malleable (or bendable), because the mobile electrons allow the atoms to slide past one another. This also allows them to be ductile (meaning they can be drawn into wires). Electric current, which is made up of moving electrons, flows freely through metals because of their delocalized electrons. -
0
Substitutional Versus Interstitial Alloys
Whenever other atoms are added to pure metals, an alloy is formed. Alloys have different properties than the pure metal they formed from. There are two major types of alloys: substitutional and interstitial. Substitutional alloys form when one of the in the structure of the metal is removed and another atom is put in it’s place. The original atom is substituted by a different atom. Bronze is an example of a substitutional alloy made up mostly of copper atoms with tin atoms occasionally substituted into the atomic pattern. Interstitial alloys form when other atoms fit into the gaps within the structure. The original pattern of atoms is maintained with new atoms inserted between the parent atoms. Steel is an interstitial alloy made up mostly of iron with carbon atoms inserted into the gaps in the structure. The sizes of the atoms involved can be helpful when predicting what type of alloy will form. In substitutional alloys, the atoms involved are roughly the same size. In interstitial alloys, the inserted atoms tend to be much smaller than the original atoms.